Extracorporeal shockwaves (ESW) are pressure waves generated acoustically. Shockwaves are different from other acoustic waves because they are at a lower frequency. There are many examples of these sonic pulse waves in real life. A good example is the sound of thunder following the flash of lightning. A more day to day version of a shockwave are those created by clapping. One of the most dramatic examples of shockwaves and their effects is an airplane breaking the sound barrier which generates an audible bang and can lead to the breakage of glass even in distant areas.
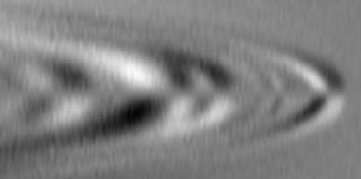
Shockwaves travel nearly unchanged through fluids, and hence soft tissues of the body, exerting their effects only where there is a change in acoustic impedance along their path.
When clinically effective shockwaves enter the body they have been described as creating ‘controlled explosions’ (Ogden et al, 2001). However, more correctly, when a shockwave enters living tissue it will either be reflected, refracted, transmitted or dissipated, just like any other wave. Therapeutic ESW energy is released at these interfaces of different impedance values. What that means is that where there is a change in structure part of the shockwave energy will be released and create compression and shear loads on the surface of the material with the greater impedance. This rapid interaction between compression and shear forces results in what is commonly referred to as cavitation. Here microscopic gas bubbles are built up on the surface of the material (the one with the greater impedance) and the collapse of these bubbles creates a small jet of liquid (fast flowing) that causes high local stress. Sturtevant (1996) believes cavitation is responsible for the effects of ESW.
Technically, shockwaves are characterised by positive pressures of up to 100 megapascals (MPa). This is debated in the literature as according to Ogden et al (2001) typical peak pressures are 50-80 MPa, although Speed (2004) described pressures between 35-120 MPa. This pressure will rapidly fall to a negative pressure of about 5-10MPa (1MPA is about 10 times atmospheric pressure). The shockwaves build up very rapidly with a rise time of 30-120 nsec and their pulse duration is 300-500 nsec (Sturtevant, 1996).
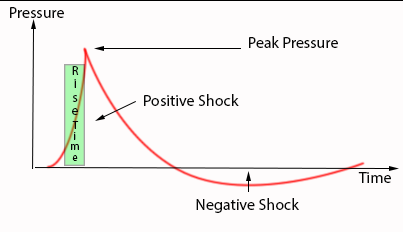
The pulse energy needs to be focused in order for it to be effective. The beam created is normally narrow, being 2-8mm in diameter. In theory, pressure and energy are concentrated at a point – the focus – which has finite dimensions. The pressure field at it’s highest is known as the focal center, but the pressure will have decreasing values in neighboring areas as well. There are 3 factors deciding this.
- -6dB-area. This is the boundary around the focus where the pressure has decreased to half of it’s peak value. It is measured in mm along the x, y and z axes.
- -5MPa-area. This is where the boundaries of focus are defined as the pressure having decreased to 5MPa. It is measured in mm along the x, y and z axes.
- 5mm-area. The focal area is simply 5mm spherically around the focus.
You will see any of the methods used by manufacturers to describe the focal region of a machine.
There are 2 fundamentally different types of shockwave (McClure & Merritt, 2003).
- Non-Focused also called Radial or Dispersive (Soft shockwave)
- Focused (Hard shockwave often referred to as lithotripsy)
Non Focused/Radial/Dispersive/Soft Shock
This web site concentrates on non-focused or radial or dispersive shockwave which is created by mechanical concussion that creates expanding wave fronts away from the concussion plate (the end of the machine) which travel forward into the body. These waves rapidly attenuate after their generation (Ammendolia et al, 2001, McClure & Merritt, 2003).
These forms of shockwave are often described as soft shock.
Radial shockwaves are generated in 2 main ways.
1. Air compressed system. Here a bullet in a barrel is accelerated using compressed air to strike an end plate generating the shockwave. These machine are characterised by requiring an air compressor and their shockwave generation is normally referred to in terms of pressure e.g. 3.0bar.
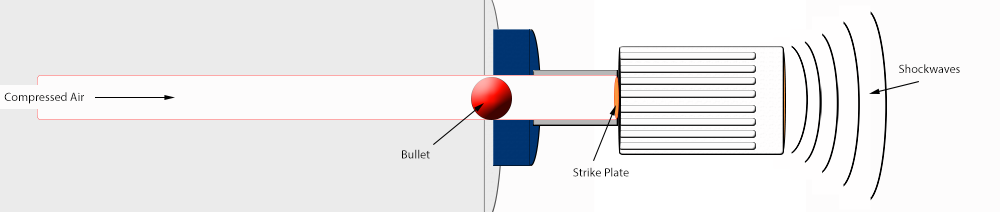
2. Electromagnetic. Here a bullet in a barrel is accelerated using alternating magnetic fields causing the bullet to strike an end plate creating the shockwave. These machines are characterised by their shockwave energy being described in terms of actual energy in milijoules e.g. 120mJ.
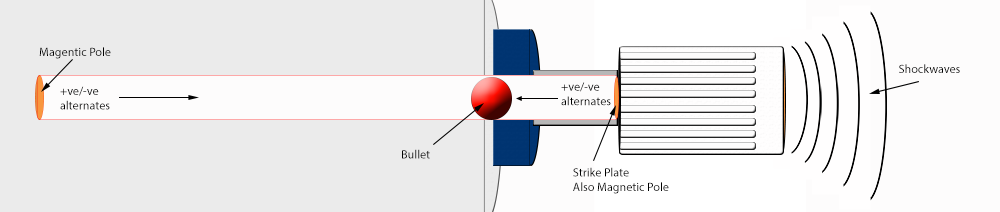
As the way shockwaves are produced differs fundamentally the measurements used to describe the energy they produce also differs. Below is a chart showing approximations of the energy delivered:
Compressed Airbar | MagneticmJ | Energy per mm using 15mm headmJ/mm2 |
1 | 60 | |
2 | 90 | |
3 | 120 | 0.12 |
4 | 150 | |
5 | 180 | 0.38 |
Shockwaves overall are divided in terms of their energy content and despite some controversy Rompe et al (1998) have described the following generally accepted groups.
- Low – up to 0.09mJ/mm2
- Medium – up to 0.28mJ/mm2 (some papers have described higher values than this)
- High – over 0.6mJ/mm2
Radial or soft shock waves are characterised by lower wave energies, slower rise time and a negative component that is of the same magnitude as the preceding positive component (McClure & Merritt, 2003) or an even larger negative component as shown by Cleveland, Chitnis and McClure (2007).
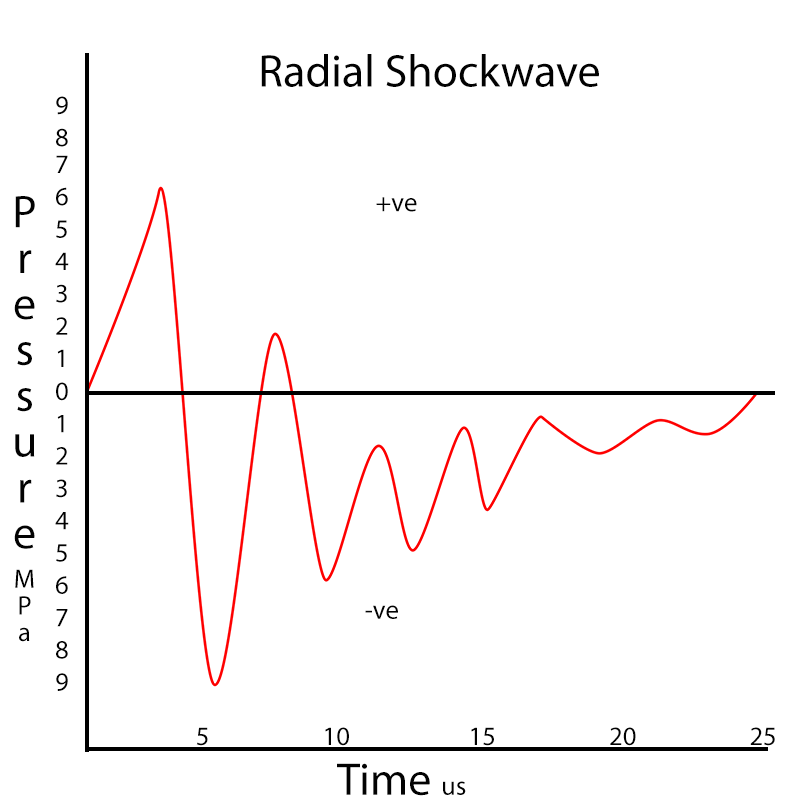
Adapted from Cleveland, Chitnis and McClure (2007).
Radial shockwave is often referred to as non-focused shockwave therapy as it is not reflected or redirected towards a focal point in the patient’s body. This means radial shockwave energy declines rapidly in proportion to the distance from the site of the generation (the end of the gun). Or in other words, the shockwaves loose power the deeper they go into the body. In the example below, a convex 15mm shockwave head delivers it’s energy across the face of the head radially (in other words it does not spread out very much). At 3.0bar (120mj) the axial penetration (depth it goes to) is 3.9cm (39mm). The treatment depth or effective distance for therapeutic purposes could be said to be the Rayleigh wave distance (mid distance from the treatment head). Further than the mid distance the waves loose so much energy they are no longer effective (Cleveland, Chitnis and McClure 2007).
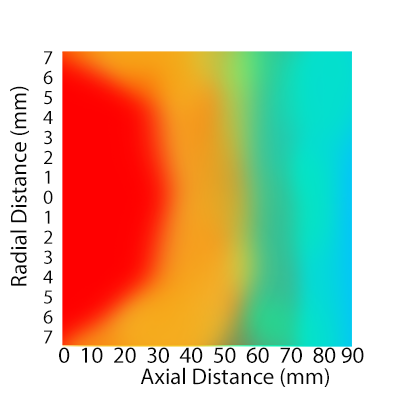
Adapted from Cleveland, Chitnis and McClure (2007).
Using a hand held applicator these waves are transmitted up to 35mm into the patient’s body (Ammendolia et al, 2001) or 39mm in water (Cleveland, Chitnis and McClure, 2007).
The depth a concussive shockwave can reach depends on the force at which the shockwave is produced (Cleveland, Chitnis and McClure, 2007). The higher the input of energy the deeper the shock will travel and the more energy will be transmitted into the tissues. Generally the shockwave produced is believed to travel out from the head of the machine in a cylindrical shape with very little energy outside of the physical size of the head i.e. a 15mm round head will give a 15mm round shock wave which will stay the shape of the head as it enters another medium (the body) (Cleveland, Chitnis and McClure, 2007).
Below are 3 common examples of force and depth using a 15mm round head with a 0.7mm convex strike plate (Cleveland, Chitnis and McClure, 2007):
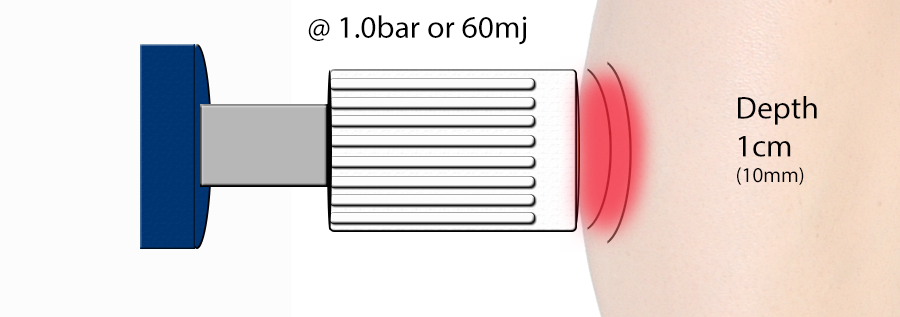
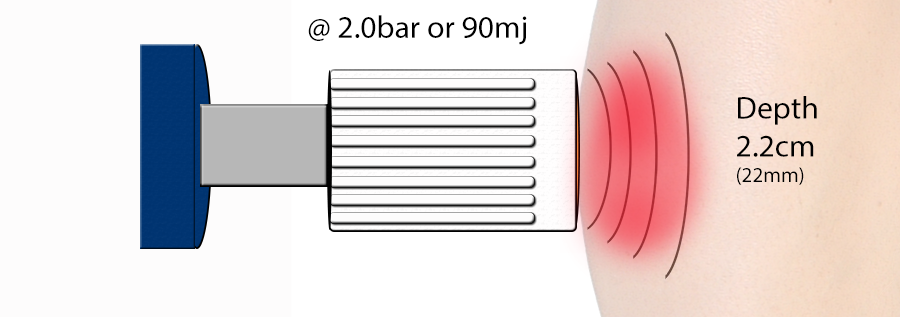
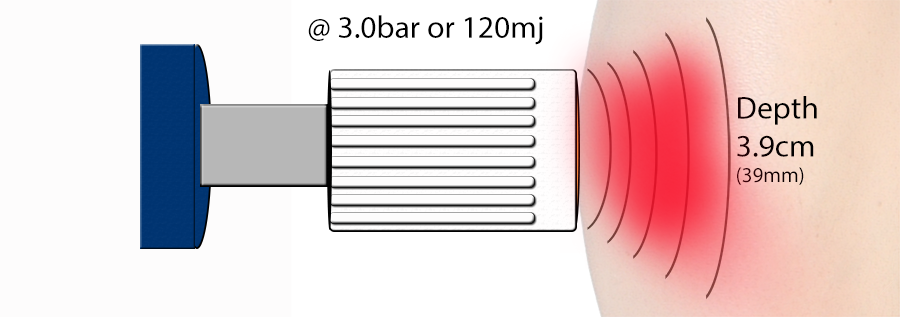
Head Size and Shape:
Radial shockwave is produced across the face of a shockwave head. These heads vary in size and shape. The most commonly used heads are 15mm across and are described as convex (the head reaches a peak in the center which is higher than the edges). A head with a physically smaller diameter will give more energy at the same power setting than a head with a bigger diameter e.g. a 30mm head will deliver 60mj (1 bar) across a wider area thus spreading the force compared to a 15mm head. A 6mm head will deliver all of the 60mj into a smaller space hence the energy level in that space will be higher. For this reason you will often see the power of the shockwave expressed as mj/mm2.
Radial shockwave heads are designed to deliver the force into a specific area (usually just in front of the head and for the width of the head). The convex shape helps with this by focusing the shockwave (not to be confused with actual focused shockwave where the point of most force may be tens of centimeters away). To improve the depth radial shockwave reaches manufacturers change the ‘face shape’ (the actual contact shape of the head) to gain ‘focus’.
An example of a normal 15mm (0.7mm convex head) can be seen below:
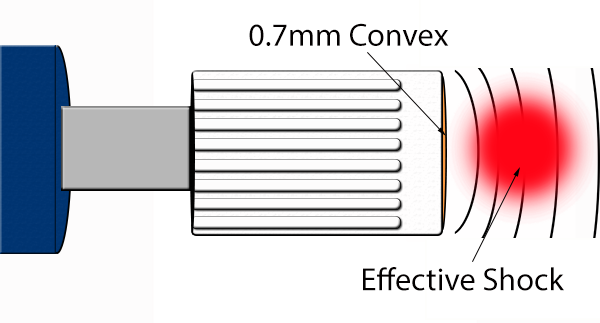
Whereas a concave head should allow some focusing of the energy as seen below:
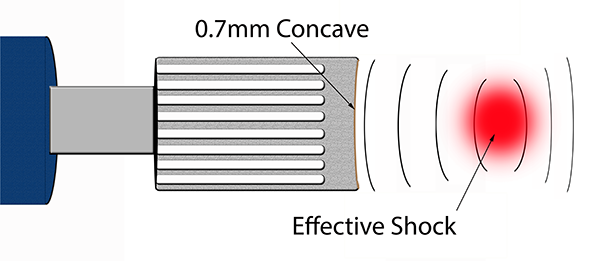
This means the depth at which the highest amount of energy applied can be varied. The area of most energy is referred to as the energy flux area.
Frequencies in Shockwave Treatment:
The effect of changing the frequency of shockwave therapy know as the pulse rate frequency (or PRF for short) may be more than just for comfort. Higher frequencies 14+hz are described by patients as feeling easier. Lower frequencies 8-12hz are often described as feeling more painful. Authors have suggested using higher frequencies at the beginning of treatment to familiarise or acclimatise the patient to the sensation then work down to the desired frequency in stages e.g. 200 shocks at 16hz then 200 at 14hz then 200 at 12hz aiming for 10hz in the end for the desired 2000 shocks.
Huber, Jöchle and Debus (1998) have shown that low frequencies (0.5hz-5hz) cause much less cavitation during treatment (there is little effect on cavitation bubble lifespan or size) whereas high frequencies (above 5hz) cause many more cavitations which increase in number with increasing speed/frequency. However, it must be noted that although increasing frequency increased the number of cavitations it did not increase the size of those cavitations; only pressure (energy delivery per mm) can do that.
Increasing frequency affects the depth of wave penetration (as lower frequency waves travel further than higher frequency waves). As can be seen below high frequency waves travel less distance (below are depictions of different wave frequencies on a gold film, adapted from Telford, Geldart and Sheriff, 1990).
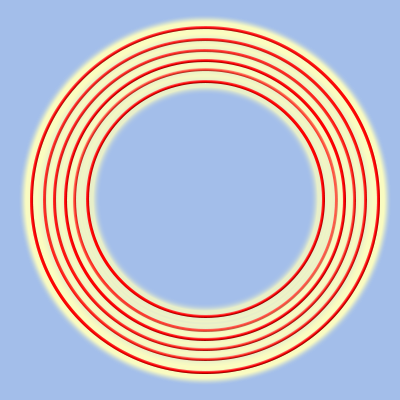
High frequency waves = less penetration
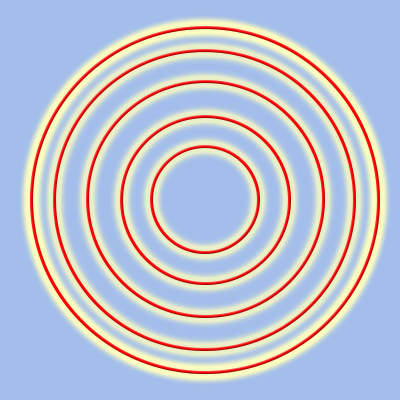
Lower frequency waves travel further
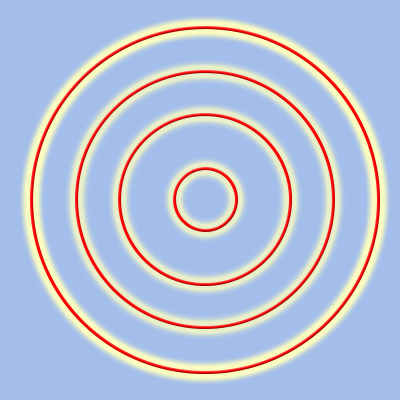
Much lower frequency waves travel distance much more easily
So increasing frequency can be used to decrease treatment depth whilst maintaining shock energy (you can create stronger effects at lower depths by increasing both the frequency and the power).
Overall Energy:
If you take the shock head size and the amount of energy given to the shock (mj/mm2 or bar) and then add in the total number of shocks given you end up with an equation which gives you the total energy applied to the tissues (e.g. 0.18mj/mm2 X 2000 shocks = 360mj/mm2). This is referred to as total energy density.
Recently manufacturers have suggested that shock wave at very high frequencies can overcome the effects of the golgi tendon organs and hence make a muscle relax more. However, as far back as 1979 Gregory and Proske described a firing rate of 174 per second for the golgi tendon organs which would mean a machine would have to have a shock rate higher than this for this principle to be true. Shockwave above 15hz is able to reduce muscle tone, but anywhere from 15-30hz will do it according to Nazarov & Gorozhani (1988).
Non-focused ESW differs considerably from focused ESW and the term shockwave may be inappropriate according to Schnewlin & Lischer (2001). However, there have been many beneficial effects of this type of shockwave therapy in various types of orthopaedic disorders in both humans (Gremion et al, 2001, Lohrer et al, 2001 a & b) and in animals (Boening et al, 2000, Palmer, 2002). Although most people focus on the human studies the animal studies may be more intriguing as there are no psychological factors involved with animal treatment processes (there is no placebo effect).
Effects of Shockwaves:
Radial shockwave effects fall into three broad categories:
- Destruction of tissue
- Healing (increased)
- Decrease of pain
Although the settings of the machines vary for each type of effect required, a description of these settings can be seen here, each shock wave will have some of each of these effects around each wave i.e. a shockwave at the highest settings (to create damage) will always have an area around the wave where there will be less energy and hence some of the other effects. An example of this can be seen in the positive part of the radial shockwave below:
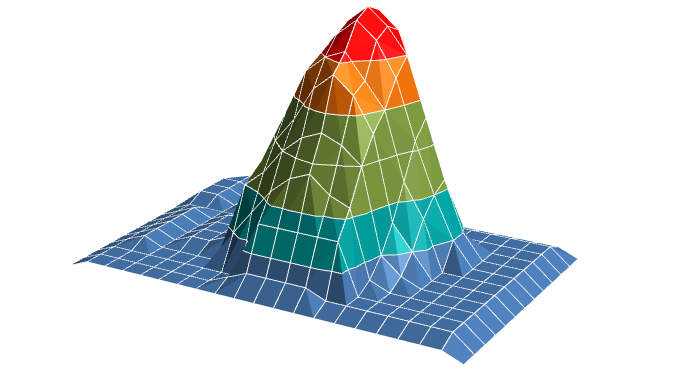
Imagine the red is the area of energy that will create damage. This area goes from the bottom of the shock to the top as a column damaging everything in it’s way. Around this column is an area of less energy, seen in green, which would be of a dosage able to create healing. There would also be an area, shown in blue, of much less energy able to decrease pain. There is also an area where the wave force would decrease above the area of damage (the energy can’t continue forever) which in turn would have healing or pain relieving effects. It needs to be remembered that the effects happen at high to low energy levels i.e. high levels = damage + healing + pain relief. Middle levels = healing + pain relief. Low levels = pain relief only. The actual effects of radial shockwave can be seen in the sections below:
Biological effects of radial shockwave can be seen here.
Tissue effects of radial shockwave can be seen here.
Focused shockwave or high energy (section for information only – this web site is dedicated to radial shockwave):
High energy shockwave has been shown to have detrimental effects on soft tissues. It normally requires some form of local analgesia to tolerate the treatment although there have been studies demonstrating that the use of local analgesia can be a negative factor to the outcome of shockwave. High energy shockwave is unlikely to ever be used in the therapy setting where most clinicians have low energy shockwave machines.
Focused shockwaves are directed by reflection at target zones into a patient’s body (Sturtevant, 1996). Focused ESW is created from a wide arc so that at the site of actual wave generation the amount of energy dispersed is minimal. This limits damage at the skin and to underlying soft tissues. The focusing mechanism directs each shock to a defined point within the body. This concentrates the energy at this point to an extremely high level. The location within the body is adjusted by altering the energy output (voltage) of the machine or by adjusting the focusing mechanism (Sturtevant, 1996). In static ESW machines diagnostic imaging is most commonly used to exactly localise the point of energy concentration and hence where the damage will be. Fluroscopy, xray or ultrasound are usually used. Creating a focus point minimises surrounding soft tissue and organ damage because the maximal (therapeutic) energy is only at the focal point (Schnewlin & Lischer, 2001). The energy at the focused point is called energy flux density and is normally recorded in energy per surface area units (mJ/mm2) (Sturtevant, 1996).
Focused shockwaves are generated by initiating a pressure wave, normally within fluid, and there are 3 different ways to do this currently.
- Piezoelectric systems. Here a crystalline material has high voltage electricity passed through it which forces it to expand or contract and hence initiate a pressure wave into the surrounding fluid.
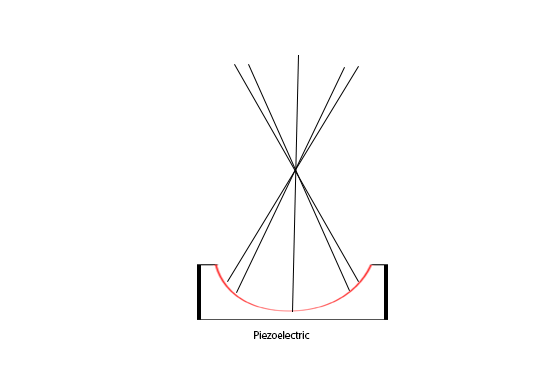
- Electromagnetic systems. Here a coil creates opposing magnetic fields when current is applied. This causes a submerged membrane to move and generate a pressure wave in the surrounding fluid medium.
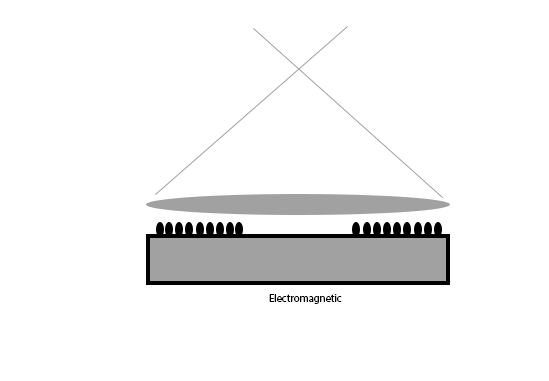
- Electrohydraulic systems. A high voltage spark creates a plasma bubble. Expansion of the bubble compresses the surrounding fluid medium which creates a pressure wave.
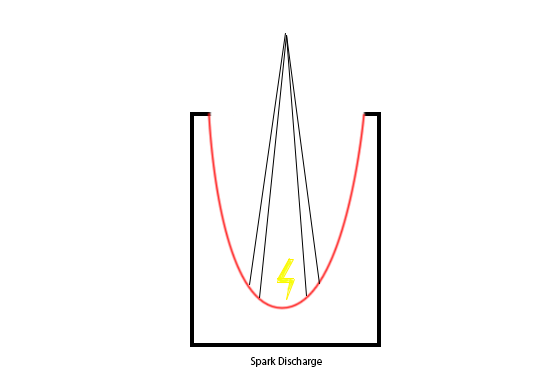
Although all 3 mechanisms create different wave forms and hence energy characteristics they all seem to be sufficient to destroy human tissue (Sturtevant, 1996).